[An Introduction To Vibration Analysis – Part 3]
Modal analysis is one of the most widely adopted vibration measurement techniques for studying the dynamic behaviour of structures and mechanical components. It allows engineers to identify a system’s natural frequencies, mode shapes, and damping ratios. In real-world applications, modal analysis is often used to troubleshoot operational issues, validate designs, and optimize performance. This post will delve into how modal analysis is conducted in the field. It will focus on comparing experimental and operational methods, testing methods like hammer and shaker tests, and shed light on their practical applications through various case studies.
Operational Modal Analysis (OMA) & Experimental Modal Analysis (EMA)
Modal analysis can generally be categorised into Experimental Modal Analysis (EMA) and Operational Modal Analysis (OMA). These two methodologies offer distinct advantages and constraints that make them suitable for different projects. Understanding the specific benefits and drawbacks of each is critical for deciding which approach to use for your specific situation.
Experimental Modal Analysis (EMA) Tests
EMA involves the application of a known, controlled excitation to the structure or component under investigation. This excitation is usually applied using an impact hammer or a shaker.
Key Features of EMA
- Provides higher accuracy because of the controlled environment.
- Suitable for smaller structures or individual components where it is feasible to apply a known force.
- Relating the measured frequencies and mode shapes to the input force is easier, providing more direct insight into the system’s dynamic properties.
Hammer Tests
The impact hammer test is one of the simplest yet most effective vibration measurement techniques for conducting modal analysis. It involves striking a test object with a force impulse provided by a calibrated hammer and measuring the resulting vibration using accelerometers.
Equipment
You would need a calibrated impact hammer, an accelerometer, and a data acquisition system for hammer tests. The accelerometer is typically attached to the object under investigation at a point where the response needs to be measured.
Advertisement
COMPLEXITY MADE SIMPLE
From sensors to DAQ to analysis & reporting, Prosig supports your entire measurement chain

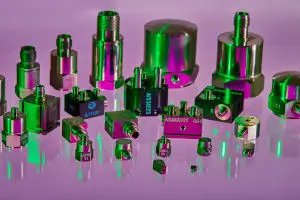
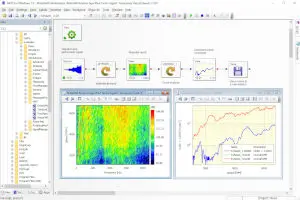
Whether you need accelerometers from our colleagues at DJB Instruments, microphones, pressure sensors or something else, Prosig can supply them as part of your system. Or you can use your own. Discover more about the Prosig hardware and software range.
Procedure
- Attach the accelerometer to the test object.
- Strike the object with the calibrated hammer.
- Record the acceleration response through the data acquisition system.
- Conduct a Fourier Transform to convert the data into the frequency domain.
- Identify peaks in the frequency response function (FRF) that signify natural frequencies.
Case Study: Automotive Suspension System
In one real-world application, engineers from an automotive company were tasked with troubleshooting a newly designed suspension system showing signs of excessive vibrations at certain speeds. A hammer test was conducted, striking various parts of the suspension assembly while recording the vibrations. Peaks were identified in the frequency spectrum that matched the speeds at which problems occurred. This led to redesigning a suspension component that successfully mitigated the vibration issues without the need for costly and time-consuming simulation processes.
Shaker Tests
Shaker tests are another common method for conducting modal analysis, offering a more controlled way to excite the system. An electrodynamic shaker applies a known force to the structure in shaker tests, and accelerometers record the response.
Equipment
Required equipment includes an electrodynamic shaker, accelerometers, and a data acquisition system. The setup often also includes an amplifier to control the shaker and a signal generator to produce the input force.
Procedure
- Attach the electrodynamic shaker to the structure at a point where force will be applied.
- Place accelerometers at various points where the response will be measured.
- Apply a known force using the shaker, which is typically a sinusoidal or random signal.
- Record the acceleration response at each accelerometer.
- Transform the recorded data into the frequency domain and identify the system’s natural frequencies, damping ratios, and mode shapes.
Case Study: Aerospace Component Analysis
In an aerospace application, an engineering team was tasked with evaluating the structural integrity of a wing component under varying loads and frequencies. A shaker test was set up to apply dynamic forces mimicking real-world operating conditions. The results clearly identified the natural frequencies and corresponding mode shapes, allowing the team to make informed decisions about material selection and structural reinforcements. This real-world test provided valuable insights that were immediately actionable, reducing the need for extended computational simulations.
Case Study: Gearbox Analysis
In a manufacturing scenario, EMA was used to analyze the vibrations in a new gearbox design. By using a controlled force input from an electrodynamic shaker, engineers were able to pinpoint the specific resonances causing inefficiencies in the gearbox. These insights led to a redesign that eliminated the problematic resonances, thereby optimizing performance.
Advantages and Limitations of EMA
Advantages
- Hammer and shaker tests are straightforward and can be conducted with basic equipment.
- They are excellent for troubleshooting and can quickly identify issues in newly designed or existing systems.
- Real-world testing can reveal phenomena that may not be captured in simulations.
Limitations
- These methods are generally limited to simpler structures; complex geometries may require more advanced techniques.
- Interpreting results requires expertise to ensure identified modes are not artefacts or noise.
Operational Modal Analysis (OMA) Tests
Operational Modal Analysis (OMA) is one of the key vibration measurement techniques and is gaining increasing attention for its ability to analyze systems under actual operating or ambient conditions. Unlike traditional modal analysis, which usually requires the system to be subjected to known, controlled excitations, OMA capitalizes on the fact that systems in operation are naturally excited by ambient forces such as wind, traffic, or operational machinery. This makes OMA particularly attractive for testing large structures or systems that are not easily accessible or for which it is impractical to create a controlled testing environment. Below we explore OMA tests in detail, touching on the equipment, procedures, and case studies that make this approach invaluable for field engineers.
[Article – An OMA technique – What is Operating Deflection Shapes (ODS) Analysis?]
Key Features of OMA
- There is no need to artificially excite the system.
- It is ideal for large structures like bridges, buildings, and wind turbines, where applying a controlled force is impractical.
- Relies heavily on advanced signal processing techniques to separate the contribution of multiple inputs.
Equipment Used in OMA Tests
The equipment setup for OMA testing usually includes:
- Multiple accelerometers: To measure the dynamic response at different points on the structure.
- Data acquisition system: To collect and store the measurement data for subsequent analysis.
- Signal processing software: To analyze the data in the frequency domain and extract modal parameters.
Procedure for Conducting OMA Tests
- Preparation and Planning: The first step involves identifying the critical points on the structure where accelerometers will be placed. Depending on the complexity of the structure, this could be a handful or hundreds of points.
- Sensor Installation: Accelerometers are mounted at the pre-identified points to measure the system’s dynamic response.
- Data Acquisition: Once the accelerometers are in place and calibrated, data collection begins. This involves recording the vibrations of the structure over a period of time, often while the system is in operation or subject to ambient conditions.
- Data Analysis: After the data are collected, they are subjected to various signal processing techniques, such as Fourier transforms or spectral density estimations, to convert the time-domain data into the frequency domain.
- Parameter Identification: Once in the frequency domain, algorithms are employed to identify the modal parameters, such as natural frequencies, damping ratios, and mode shapes.
- Interpretation and Recommendations: The last step is to interpret the identified modal parameters in the context of the structure’s operation and make any necessary recommendations for design modifications or maintenance actions.
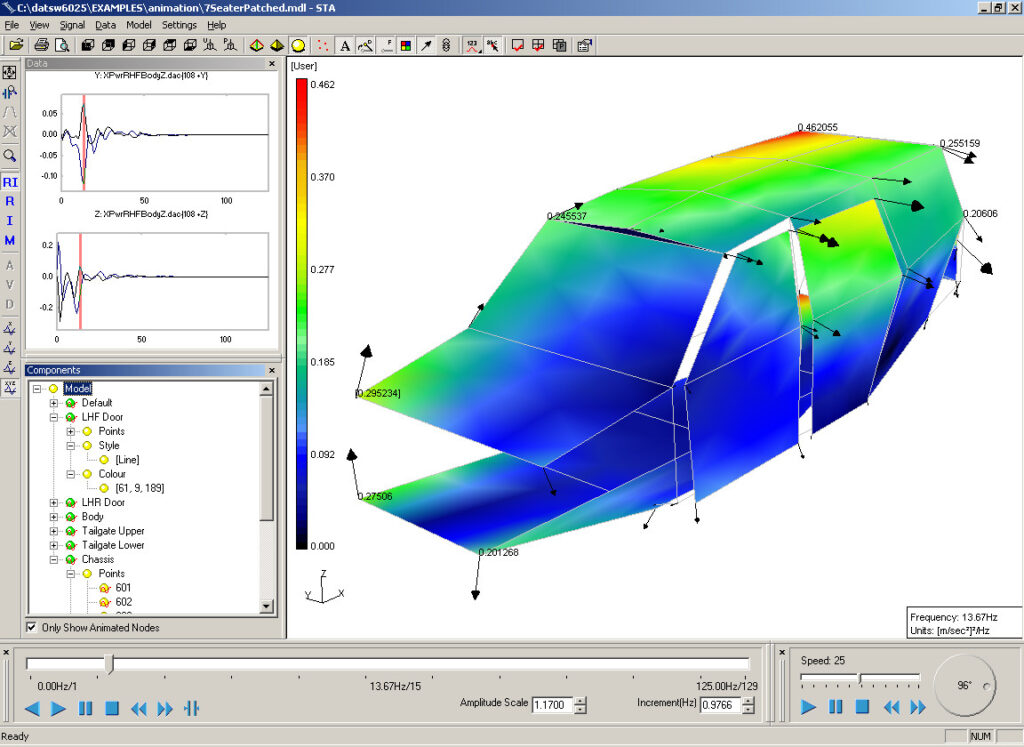
Case Studies on OMA
Case Study: Wind Turbine Monitoring
One of the more compelling applications of OMA is in the renewable energy sector, monitoring the health of wind turbines. Given the impracticality of halting a turbine for testing or applying controlled excitations, OMA proves indispensable. Engineers were able to identify a critical change in the damping ratios over time, signalling wear and tear that could lead to catastrophic failure if not addressed.
Case Study: Building Structural Health
In the civil engineering domain, OMA is commonly used for assessing the health of buildings, especially after significant events like earthquakes. By comparing the modal parameters before and after the event, engineers can make accurate assessments of the building’s structural integrity.
Case Study: Railroad Bridge Monitoring
OMA was employed to assess the health of a railroad bridge that had been in service for several decades. Here, the ambient excitation was provided by the regular train traffic over the bridge. By constantly monitoring the natural frequencies and other parameters, engineers were able to detect the signs of material fatigue and recommended timely maintenance, thus preventing potential failure.
[Article – an example of OMA testing – Tools for motorcycle dynamics analysis]
Advantages and Limitations of Operational Modal Analysis (OMA)
Operational Modal Analysis (OMA) has carved out a niche in structural and mechanical engineering due to its unique approach to modal testing. Here’s a rundown of the advantages and limitations of OMA:
Advantages
- Real-world Conditions: One of the most significant advantages of OMA is its ability to test systems under actual operating conditions, which can provide more realistic results.
- No Controlled Excitation Needed: Unlike Experimental Modal Analysis (EMA), OMA does not require the application of a known, controlled force, making it convenient and less intrusive.
- Versatile: OMA can be applied to a broad range of systems, from small mechanical components to large structures like bridges or buildings.
- Less Expensive: Since it doesn’t require specialized equipment to apply a controlled force (like shakers or impact hammers), OMA can often be less expensive to implement.
- Unobtrusive: OMA can often be performed without interrupting the normal operations of a system or structure, making it ideal for monitoring over time.
- Good for Difficult-to-Access Systems: In scenarios where it is logistically challenging to apply controlled excitations, like offshore wind turbines or high-rise buildings, OMA is often the only feasible option.
Limitations
- Data Complexity: OMA relies on ambient or operational excitations that are random and unmeasured, making the data more complex to analyze.
- Limited Control on Excitation: The lack of control over the nature and magnitude of the excitations can sometimes make it difficult to excite specific modes of interest.
- Ambiguity in Results: Since the forces causing the vibrations are not measured, there is often less certainty in the identified modal parameters, compared to EMA.
- Extended Data Collection: To obtain reliable results, extended periods of data collection may be required, which can be a limitation in some scenarios.
- External Noise: The presence of other ambient forces or operational conditions can introduce noise into the data, requiring more advanced signal processing techniques for accurate results.
Understanding the advantages and limitations of OMA can help engineers make informed decisions about when to employ this methodology, ensuring that it is used in contexts where its unique strengths are most beneficial, and its limitations can be appropriately managed.
Summary of OMA testing
OMA stands as a robust tool for engineers interested in capturing the dynamics of a system under its actual operational conditions. Its power lies in its ability to offer valuable insights without the need for controlled, artificial excitation, making it highly versatile and suitable for a range of applications. Through careful planning, precise measurement, and thoughtful interpretation, OMA can provide critical information to ensure the long-term reliability and safety of various structures and mechanical systems.
Comparing OMA and EMA
Here’s a quick comparison to help you decide which approach suits your needs:
EMA:
- Controlled but often limited to lab settings or smaller systems.
- More straightforward data interpretation.
- Requires controlled excitation (e.g., impact hammer or shaker).
OMA:
- More suited for real-world, in-situ testing of large or complex structures.
- Data interpretation can be more complex due to the unmeasured, ambient excitation.
- No need for artificial excitation, making it more convenient for certain applications.
Summary OMA and EMA
Both OMA and EMA have their places in the realm of modal analysis. EMA, with its controlled environment, offers precision but may be restricted by the size and complexity of the system. OMA, on the other hand, offers the flexibility to assess larger and more complex systems in their natural operational settings but may present challenges in data interpretation. Through understanding the merits and limitations of each, engineers can make informed choices about which modal analysis method best suits their specific application.
Conclusion
Modal analysis is one of the core vibration measurement techniques and remains a cornerstone in the toolbox of vibration engineers, providing invaluable data on the dynamic behaviour of systems. In real-world applications, hammer and shaker tests offer straightforward, quick, and often cost-effective means to gather this essential data. These simple testing methods have been proven to offer actionable insights in various industries, as illustrated by our case studies in the automotive and aerospace sectors.
Some Recommended Reads:
- “Modal Testing: Theory, Practice, and Application” by D. J. Ewins : A comprehensive guide covering both the theoretical and practical aspects of modal testing.
- “Mechanical Vibration and Shock Analysis” by Christian Lalanne : An exhaustive multi-volume set that delves into various types of mechanical vibrations and shocks.
- “Fundamentals of Structural Dynamics” by Roy R. Craig & Andrew J. Kurdila : A foundational text offering both theoretical and practical insights into structural dynamics, including modal analysis.
- “Random Vibrations: Theory and Practice” by Paul H. Wirsching, Thomas L. Paez, and Keith Ortiz : Focuses on the statistical aspects of vibration analysis, relevant for engineers dealing with random forces.
See the whole Basic Signal Processing series
Feedback and discussions are encouraged in the comment section. Dive deep, analyze thoroughly, and remember – in every oscillation, there’s information waiting to be decoded.
Latest posts by Chris Mason (see all)
- Rotating Machinery Vibration Analysis: Keeping Your Machines Humming Along - November 27, 2023
- Vibration Control and Isolation: A Comprehensive Guide for Engineers - November 6, 2023
- Modal Analysis in the Real World - October 6, 2023